Let’s take a brief look at resources available on the moon and what they mean in terms of a growing system in place and aspects which might be interesting in the context of Plan0. Just fast recap.
Gravity
1/6 of earth(1.62m/s^2), escape velocity 2.38 km/s
Energy
Energy wise things aren’t too good but aren’t bad either. At the peak, the sun provides about 1360W/m2 of energy, but there are night and day in most parts of the celestial body, and the same for earth equator is the sunniest place, and the peak of energy for non-tracking setup is at noon.
Day on the moon is 29.5/2 earth days. And average energy received by the system during a day is about 0.63 of peak power(2/pi). Things can be better for a system which tracks the sun, but it comes with the need to separate collectors by some distance for them to do not cast shadows on each other. If we average the energy with the night time it 2 times less about 0.31 of peak power or 1/pi.
The temperature on the surface about – day max 120 °C, night min -150 °C.
The temperature at 1m from the surface is about -35 C day/night and isn’t much affected by surface temperatures.
Surface
Regolith layer about 6m deep(3-12m deep in Apollo landing sites) – loose dust on the surface, packed dust when it goes deeper.
Concentration and size of big rocks on the surface varies significantly.
Particle sizes of the regolith dust vary from few millimeters size to micron sizes.
It looks like that:
A good moon rover shot, to see how it behaves under a vehicle.
There is some additional information about sizes of particles here, more than half of particles in the soil are less than 0.1mm in size, and a significant percentage is a fine dust of 0.04mm size or less(about 18% by mass)
Interesting numbers of potential impactors per square meter per year, from the same source – about 30000 impacts per year per square meter of surface, which produce 0.1um craters, 300 impacts produce craters 10um and more, 0.1mm and more about 0.6 impacts, 1mm and more 0.001(one impact per 1000 square meters per year).
Composition
percentages in the table are weight percentages.
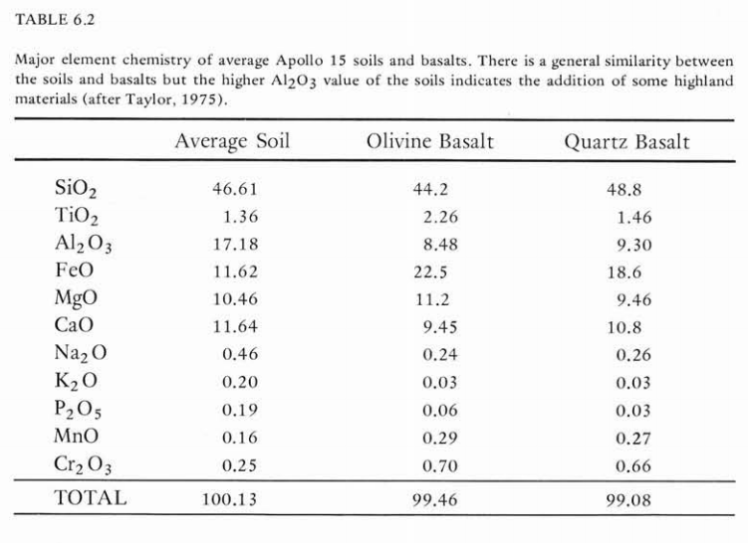
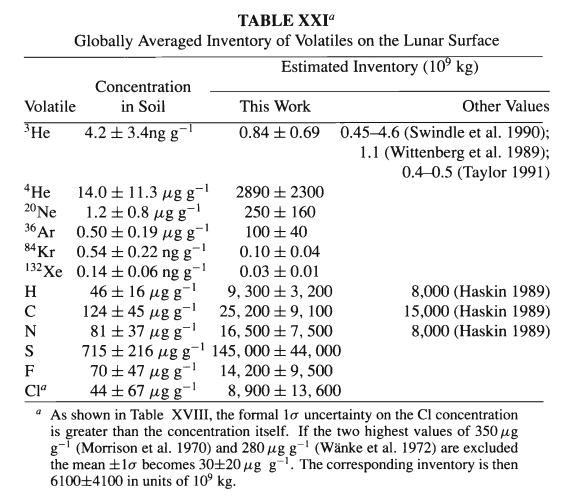
There are mentioning about reduced aka no oxidized Fe or even Al, and for Fe I have seen the numbers as 0.5 wt% of regolith is reduced iron, which might be stuck on other particles(glass and all that) as tiny particles. So to separate them you still probably need to melt that thing even after separation. But it is hard to find that information, but generally, the moon has reduction environment because of solar wind hydrogen, and high-temperature decompositions because of meteorites impacts.
But information hard to find, maybe because of most of it old and they didn’t focus them selfs at that time on ISRU.
What it gets us in terms of a Plan0 system.
Let’s produce some new data for us to think about
If we build our equipment from Al, Fe, Mg, Si, Ti we face the problem that a lot of it is in oxidized state, and the system should spend energy to convert them to the state it used to have those materials. It can be done by direct electrolysis as an example, or by fluorine extraction methods or by others, but to get those materials for further work one should spend a particular amount of energy which is determined by the enthalpy of the chemical composition, and it is the factor which can not be changed. There are other ways around the problem, but later about that, let’s assume we need those materials or at least some of them, and we use direct electrolysis, which is magically 100% efficient and other losses.
In the table of material, not all of materials are mentioned, so total wt% is about 80+%, not a 100, but it does not affect the general picture.
Different materials require different energy to produce a mole of material, a kg of material, or a cubic meter of material. And in some use cases, the strength of materials might be not that important, for example, heat storage, or heat conduction, or coating or something else and you might wish to optimize processes based on volumetric energy expenses, or in other cases by chemical properties and energy to produce a mole of material will be important, or you need a certain amount of mass, or you need a combination of properties like mass/strength. Btw more or less pure iron isn’t such a strong material which we used to associate it with in form of the steel thus it might be not such an easy case to choose it over aluminium+Mg as an example, which is easier to work with as an example(kinda).
If we need mass for some application, kinetic energy storage as an example, Iron is definitely a winner because it is the cheapest to reduce per kg or mass.
And so on.
So, let’s continue with electrolysis and let’s assume we set a goal to electrolyze about 1 ton of regolith material per hour.
With our magical electrolysis process, it requires about 3.2MW of electricity production.
To generate that electricity we need means of convert solar energy (or use a reactor for that), and those means of electricity production will use materials we extract from regolith and can be characterized by a mass of materials used to generate one kW of power.
In the table used a pretty arbitrary number of 20kg/kW, and we do not specify which of the ways we are using – solar panels, solar concentrators, reactor, combustion engines(joke, here you can laugh), thermionics or other specific ways to generate electricity. Most likely some sort of solar energy conversion. For those methods, it might be a reasonable number, based on currently available technologies for that, but is not exact, not the best etc.
Thus those 3.2MW of average power represent some amount of matter which should be used to build means which extract this power.
However, we have other factors which affect our situation, day/night for the moon as an example. A solar installation on the moon surface is not working half of the time, because of night time, sometimes it does not work at its maximum capacity because of elevation of the sun over the horizon. Thus to keep with average energy production of 24.7.365, the installation has to be bigger and have higher electricity peak production.
If we assume 0 expenses on storing the energy, basically we do not store it but just use it 100%, then the difference between those installations, one affected by day/night cycle and one not affected by them, isn’t that big, about 5 times. At least we would not count it as a significant difference, there on earth, yeah day/night so it is, so works, what you can do about that on earth.
Based on energy produced by the systems and mass of materials required, and energy required to extract those materials from regolith and excluding energy expenses to actually build things from those materials or losses of a different kind, we can estimate the time needed for the system to double its size. Any expenses and losses will make the time longer but do not affect the overall picture.
We might do those calculations assuming different prevalent materials used in the system, and based on its percentage in lunar soil and considering other materials as waste in that electrolysis process. We will get different numbers for the different combination but because of selected method of extraction(for its simplicity) obviously, we get better results when we use all materials which we get in the process. Electrolysis is just an example of a method for bulk extraction, not the best one or something like that, just an example.
All that said the difference between using mostly Iron or Aluminium isn’t significant because we get about the same amount by mass of both materials and the production capacities defined in terms of kg per kW. And in the case of the system with constant energy supply doubling the capacities happens in about 720 hours or 30 days.
With the system which faces the problems of day night cycles, the time it about 5 times more, about 150 days.
For a system where we have less waste and more fully utilize the produced stuff, times are 140 hours and 700 hours for those systems respectively.
More interesting stuff begins when we consider a situation, where we send some initial energy production of X amount of kW (10, 100, 1000) and we try to reach some landmark (1GW in the table). Land mark can be a certain amount of energy production which allows us to implement more sophisticated technological processes which require a certain amount of energy just to be able to work at a rate which justifies spending energy on them – processors production, mass driver, human colony if some one wishes it to have, more sophisticated resource separation extraction methods etc.
What do we see there? If we start with 10kW in a system which has 24.7.365 energy supply the 1GW landmark will be reached in about 500 days. In a system which faces the problems of day/night, the landmark will be reached in 2465 days. All that in cases when we use Al or Fe as main materials. In a case of a better resource utilization, those times are 95 and 480 days respectively.
However, needs to be noticed that that initial energy budget of that ideal tech seed case, does not affect the time so much. The difference between sending 10 kW system(which we assumed to be 20kg/kW) or 100 times bigger system it not that impressive in terms of achieving that energy landmark. And delivery of one system may cost $400 million and another $40 billions, with currently announced prices of $2 million per kg on the surface of the moon(by one company).
With the decent utilization of extracted resource and using Al+Mg+Fe+Ti, which we easily can imagine being construction materials for most of the parts of a system one may come-up with, the difference of reaching 1GW level of energy production is 1 year, about 1.5 and 2.5 years respectively, for a D/N system which starts with 10kW and 1000kW. So even if you have some source of income on earth which brings you $40 billion annually as pure profit(after taxes and all that) it is hard to justify spending those $40 billion on delivery of a bigger system instead of R&D the next steps of the system or improving existing one by remotely introducing better ways to do stuff in place.
Another thing to notice is that a simple system which does not track the sun too much is about 5 times slower in growth than one which has a constant supply of energy.
The best number for solar panels I have seen and remembered was 1.3 year it is required for it to return the energy which was spent on its production, in some best use case for the earth, which also has that problem of day night cycles. So moving the technology in space, let’s say Earth Sun L1, will reduce the time of EROEI also about 5 times, and it will be able to double its size once in about 3 months.
Some important moments about all this situation:
- 24.7.365 energy supply is better than day night cycle
- Initial size is not that much important, bigger is not necessarily better if it costs too much
- Law’s of Physics/Chemistry still applies and are important, no magic there
- Time required to double the size is an important parameter for such system
There are some conclusions to draw about any system of that type:
- Move the production and processing of energy costly materials to a place with 24.7.365 energy supply as soon as it is reasonable
- Use processes which require less energy to achieve sufficient results.
The second one isn’t that obvious or better to say isn’t that trivial. Obviously, it is important, but how to achieve that is a question.
First of all “sufficient result” is important there. For such a system if it requires spending 2 times more energy to achieve an increase of +5% efficiency, let’s say by producing equipment which breaks with 95% probability instead of 90% in any given time range, it will be not a good improvement for the system. It may significantly slow the system down. So, the efficiency which is a must parameter for any system on earth, and which is the focus of any development, isn’t that important parameter to optimize. But of growth of the whole system is that important parameter to optimize.
Improving or introducing a more efficient process does not necessarily mean improving the time, and should be analyzed in terms of mean by which they are happening and a number of new processes they introduce. Improvement should be considered in the context of the whole system, including means which allow those processes to happen, especially for a small system which does not host all known processes and technologies.
Using less expensive processes, which might include direct using of solar radiation instead of producing electricity.
Selective electrolysis of regolith in favor of producing Iron, as the first component which goes out of the electrolysis batch because of lower voltage required for it to be reduced.
More efficient combining the processes, because of high temperatures involved it might be possible to combine melting, volatile extraction, electrolysis, electricity generation, glassy parts production in one process.
In absence of the wind, rain etc and lower gravity(or even 0g in microgravity environment) glass is decent material to produce some structural parts to solar concentrators as an example.
Using plasma for coating for creating reflective surfaces is a good as using polish metals sheets to achieve the same results.
Same way as zinc is used to produce floating glass on earth, iron can be used to produce flat glassy surfaces from the slag from electrolysis process. Transparency or significant structural strength is not that important, but the roughness of one side of surface might be important for creating reflectors.
Even digging 3m wide and 0.5m deep trench on the surface of the moon, tramping its walls and coating it with some amount metal will create a concentrator which will work, and placing a pipe in focal point will create means to extract energy without significant expenses on creating such system. It probably won’t last long, however, the only things which will destruct it are meteorites and lunar quakes. And you achieve 2 goals while construction extracts top soil with volatiles, and create a system which serves for energy extraction process.
Everything is good as long as it reduces duplication time, and there are a lot of solutions which possible in terms of physics but impossible on earth, thus thinking about a system which grows on the moon it requires to keep in mind there are things possible which aren’t possible on earth. And those things have to be exploited. We should take advantage of them.
One of the problems of taking advantage of existing environment on the moon it that we do not have enough experimental data to create a significantly detailed plan for that. We can create some bones of the plan based on data we already know but it does not mean it will be indeed the best way of doing things, which can be tested or developed after some time of working on the moon. Thus it is important to introduce a system which we can adapt remotely, it is important to introduce a system through which we can conduct different testing.
A typical plan from NASA and others – we will send the best human and best equipment they will do things, we will R&D on earth, even more, then we will send another set of improved equipment, rinse and repeat. The main notion is that at some point in this interactions, they will be able to produce some parts locally.
One of the problems of such approaches, they initially from the start set a very high level of technologies which they would like to have. It is the same problem with human presence, it sets a very high level of required technologies. And because of the level of technologies, it does not allow to evolve it in place, because the whole technological continents are missing to be able to do so, to produce even one part of that advanced equipment. As result, you have everything or nothing. As we see on the current stage of thing it is rather nothing.
An interesting thing to read from Gregory S. Chirikjian, An Architecture for Self-Replicating Lunar Factories, pdf One of the guys which should be involved in R&D of such system, because of a good way of thinking about those matters.
And in the same way, a good example of that not that many people think about the problems a self-replicating system is facing on the moon. It is not easy to find any information about the availability of pure metals, even if they are mixed/agglutinated with glass. I remember that I have seen ISRU study of aluminum extraction from 70x or 80x on some of the NASA sites for where it was mentioned the presence of reduced aluminum particles, but unfortunately, I have lost the link, and nasa sites are just a mess. And in the Gregory S. Chirikjian’s work, he refers to one of the relatively old books about moon, which very briefly and shortly mentioning iron crystals forming on glass particles as result of evaporation from meteorites impact and further condensing. I have seen even mentioning that there is 0.5wt% of such iron present in the regolith.
Moon has a reducing environment, and those things have to be presented there. And 0.5wt% is a huge number in terms of a system which will use energy as less as possible and get building block as much as possible. Exactly a system which goal is to grow as fast as reasonably possible.
It is possible to separate such Fe with magnetic separation, even if it requires to melt the thing to extract iron, it is cheaper and easier than electrolyzing the regolith. Separating a small percentage of conductive but not magnetic material is also possible with magnetic means.
And if the system initially designed for other methods, which we know will work, and we have the ability to evolve it, and operate it remotely we can switch it to execute a new plan without sending additional stuff. But if you have an initially advanced system, and it is not big enough to produce parts of itself and evolve, we can’t switch to another plan.
As a conclusion
Moon offers a lot of good stuff, and we should creatively use what it has. A system should grow on the processes we are certain will work, but at the same time allow us to change plans on the fly to adapt it to new opportunities and discoveries. Complexity is not always the friend, same as his brother efficiency.
An interesting overview of electrolysis process of lunar regolith:
“Molten Regolith Electrolysis Reactor Modeling and Optimization of In-Situ Resource Utilization Systems Samuel S. Schreiner, Dr. Jeffrey A. Hoffman”